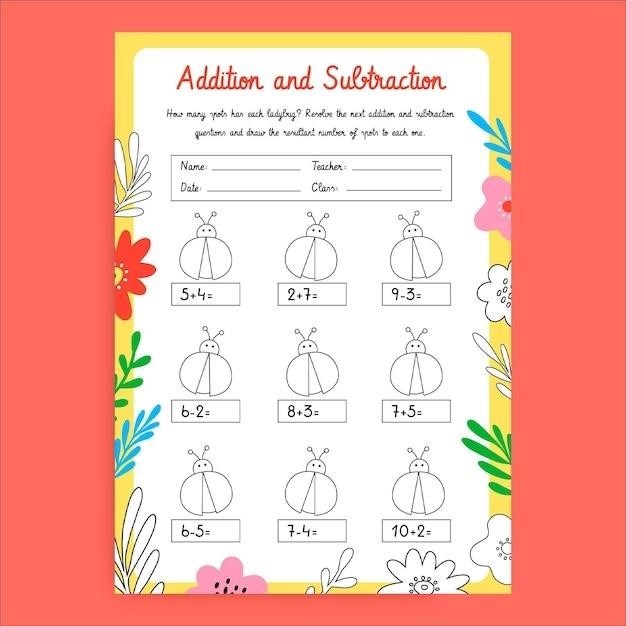
genetics practice problems worksheet answers pdf
These resources can be used to help students learn about the basics of genetics‚ including Mendelian genetics‚ Punnett squares‚ incomplete dominance‚ and sex-linked traits. They can also be used to practice solving genetics problems. Some of the resources are available as PDF files‚ which can be downloaded and printed. Others are available online‚ and can be accessed from any computer with an internet connection.
Understanding Genetics Practice Problems
Genetics practice problems are a valuable tool for students to understand the concepts of heredity and inheritance. These problems often present scenarios involving traits‚ crosses‚ and inheritance patterns‚ allowing students to apply their knowledge and develop critical thinking skills. By working through these problems‚ students can gain a deeper understanding of the mechanisms behind how traits are passed from one generation to the next.
Genetics practice problems can cover various topics‚ including Mendelian genetics‚ non-Mendelian genetics‚ Punnett squares‚ incomplete dominance‚ codominance‚ sex-linked traits‚ and pedigree analysis. Each type of problem focuses on different aspects of genetics‚ helping students develop a comprehensive understanding of the subject. For instance‚ Mendelian genetics problems explore the basic principles of inheritance‚ while non-Mendelian genetics problems delve into more complex inheritance patterns‚ such as incomplete dominance or codominance.
These practice problems can be found in textbooks‚ online resources‚ and even in worksheets designed for specific educational levels. They often include detailed explanations‚ diagrams‚ and answer keys‚ providing students with a clear understanding of the concepts and solutions. By engaging with these resources‚ students can reinforce their learning‚ identify areas where they need further clarification‚ and develop their problem-solving skills in genetics.
Types of Genetics Problems
Genetics practice problems encompass a wide range of scenarios‚ each designed to test understanding of specific genetic principles. These problems can be broadly categorized into types based on the genetic concepts they address. Some common types include⁚
- Mendelian Genetics Problems⁚ These problems focus on the basic principles of inheritance as described by Gregor Mendel. They typically involve monohybrid or dihybrid crosses‚ where students are asked to predict the genotypes and phenotypes of offspring based on parental genotypes.
- Non-Mendelian Genetics Problems⁚ These problems explore inheritance patterns that deviate from Mendel’s classic model. Examples include incomplete dominance‚ where the heterozygous phenotype is a blend of the homozygous phenotypes‚ and codominance‚ where both alleles are expressed equally in the heterozygote.
- Punnett Square Problems⁚ These problems utilize Punnett squares‚ a visual tool for predicting the possible genotypes and phenotypes of offspring from a cross. Students are often asked to construct Punnett squares and analyze the resulting ratios of genotypes and phenotypes.
- Sex-Linked Trait Problems⁚ These problems explore traits that are carried on sex chromosomes‚ typically the X chromosome in humans. Students may be asked to determine the probability of a child inheriting a sex-linked trait based on the genotypes of their parents.
- Pedigree Analysis Problems⁚ These problems involve analyzing family trees‚ known as pedigrees‚ to determine the mode of inheritance of a specific trait. Students may be asked to identify the genotypes of individuals in the pedigree based on their phenotypes and relationships.
By working through these different types of problems‚ students can gain a deeper understanding of the diverse ways in which genes are inherited and expressed.
Mendelian Genetics
Mendelian genetics‚ named after Gregor Mendel‚ the father of genetics‚ forms the foundation of our understanding of inheritance. It deals with the fundamental principles of how traits are passed from parents to offspring. Mendelian genetics problems typically focus on the following key concepts⁚
- Dominance and Recessiveness⁚ Traits are often controlled by two alleles‚ one from each parent. Dominant alleles mask the expression of recessive alleles. For example‚ in pea plants‚ the allele for tallness (T) is dominant over the allele for shortness (t)‚ so a plant with Tt genotype will be tall.
- Genotype and Phenotype⁚ Genotype refers to the genetic makeup of an individual‚ represented by the combination of alleles they possess. Phenotype is the observable characteristic or trait expressed due to the genotype. For example‚ a plant with TT or Tt genotype will have a tall phenotype‚ while a plant with tt genotype will have a short phenotype.
- Monohybrid and Dihybrid Crosses⁚ Monohybrid crosses involve tracking the inheritance of a single trait‚ while dihybrid crosses involve tracking the inheritance of two traits simultaneously. Punnett squares are often used to predict the genotypes and phenotypes of offspring in these crosses.
- Law of Segregation⁚ This law states that during gamete formation‚ the two alleles for a trait separate‚ so each gamete receives only one allele. This ensures that offspring inherit one allele from each parent.
- Law of Independent Assortment⁚ This law states that alleles for different traits segregate independently during gamete formation. This means that the inheritance of one trait does not influence the inheritance of another trait.
Practice problems in Mendelian genetics help students solidify their understanding of these basic principles and apply them to solve various inheritance scenarios.
Non-Mendelian Genetics
While Mendelian genetics lays the groundwork for understanding inheritance‚ many genetic patterns deviate from its simple principles. Non-Mendelian genetics encompasses these exceptions‚ providing a richer and more complex picture of how traits are passed down. Here are some key concepts explored in non-Mendelian genetics⁚
- Incomplete Dominance⁚ In this scenario‚ neither allele is completely dominant over the other. The heterozygous genotype results in a phenotype that is a blend of the two homozygous phenotypes. A classic example is the snapdragon flower‚ where a cross between a red (RR) and a white (rr) flower produces pink (Rr) offspring.
- Codominance⁚ Both alleles are expressed equally in the heterozygous genotype‚ resulting in a phenotype that exhibits both traits simultaneously. For instance‚ in human blood types‚ the AB blood type is codominant‚ where both A and B antigens are present on red blood cells.
- Sex-Linked Traits⁚ These traits are carried on sex chromosomes‚ typically the X chromosome in humans. Since males have only one X chromosome‚ they are more susceptible to expressing recessive sex-linked traits. Examples include color blindness and hemophilia.
- Epistasis⁚ The expression of one gene influences or masks the expression of another gene. This can lead to unexpected phenotypic ratios. For example‚ in Labrador retrievers‚ the coat color gene is epistatic to the pigment gene‚ affecting whether the dog will have black‚ chocolate‚ or yellow fur.
- Polygenic Inheritance⁚ Multiple genes contribute to the expression of a single trait‚ leading to a wide range of phenotypes. Examples include skin color‚ height‚ and intelligence.
Non-Mendelian genetics problems often challenge students to go beyond simple dominance and recessiveness‚ requiring them to understand the unique patterns of inheritance associated with these complex genetic interactions.
Punnett Squares
Punnett squares are a fundamental tool in genetics‚ providing a visual representation of the possible genotypes and phenotypes of offspring resulting from a cross between two parents. They are named after Reginald Punnett‚ a British geneticist who developed this method in the early 20th century.
Here’s how Punnett squares work⁚
- Determine the genotypes of the parents⁚ This involves identifying the alleles each parent carries for the trait in question; For example‚ if a parent is homozygous dominant for brown eyes (BB)‚ they have two B alleles. If another parent is heterozygous (Bb)‚ they have one B allele and one b allele.
- Create the Punnett square⁚ Draw a grid with the possible gametes from one parent listed across the top and the possible gametes from the other parent listed down the side. Each box in the grid represents a possible genotype of the offspring.
- Fill in the Punnett square⁚ Place the alleles from each parent’s gametes into the corresponding boxes. For example‚ if the mother’s egg carries a B allele‚ and the father’s sperm carries a b allele‚ the offspring will have the genotype Bb.
- Analyze the results⁚ Examine the genotypes in the Punnett square to determine the phenotypic ratios of the offspring. For instance‚ if the Punnett square shows that 25% of the offspring have the genotype BB‚ 50% have Bb‚ and 25% have bb‚ the phenotypic ratio for brown eyes to blue eyes might be 3⁚1 if brown eyes are dominant;
Punnett squares are a powerful tool for understanding the principles of Mendelian genetics. They can be used to predict the probability of offspring inheriting specific traits‚ and they are often used in genetics practice problems.
Incomplete Dominance
Incomplete dominance is a deviation from the classic Mendelian inheritance pattern where neither allele completely masks the other in a heterozygote. Instead‚ the heterozygote exhibits a phenotype that is a blend or intermediate between the two homozygous phenotypes.
A common example is flower color in snapdragons. If a homozygous red snapdragon (RR) is crossed with a homozygous white snapdragon (rr)‚ the offspring will all be heterozygous (Rr) and have pink flowers. This demonstrates that neither the red nor the white allele is dominant; they both contribute to the pink phenotype.
Here’s how to represent incomplete dominance in Punnett squares⁚
- Assign letters to the alleles⁚ Use uppercase letters for the dominant allele and lowercase letters for the recessive allele. For example‚ for flower color‚ you could use R for red and r for white.
- Determine the genotypes of the parents⁚ In a cross between a homozygous red snapdragon (RR) and a homozygous white snapdragon (rr)‚ the parents’ genotypes are RR and rr‚ respectively.
- Fill in the Punnett square⁚ Place the alleles from each parent’s gametes into the corresponding boxes. Since both parents are homozygous‚ all offspring will be heterozygous (Rr).
- Analyze the results⁚ The Punnett square shows that all offspring have the genotype Rr. Since incomplete dominance is in play‚ the phenotype of the offspring will be pink‚ a blend of red and white.
Understanding incomplete dominance is essential for accurately predicting offspring phenotypes in situations where neither allele is fully dominant. It expands our understanding of inheritance patterns beyond the simple dominant-recessive model;
Codominance
Codominance is another variation on Mendelian inheritance where both alleles in a heterozygous genotype are expressed simultaneously‚ resulting in a phenotype that displays both traits. Unlike incomplete dominance‚ where the phenotype is a blend‚ codominance leads to a phenotype that exhibits both alleles distinctly.
A classic example is the AB blood type in humans. The alleles for blood type are represented by IA‚ IB‚ and i. IA and IB are codominant‚ meaning that if an individual inherits both IA and IB alleles‚ they will express both A and B antigens on their red blood cells‚ resulting in the AB blood type.
To understand codominance in Punnett squares⁚
- Assign letters to the alleles⁚ Use superscripts to distinguish the codominant alleles. For example‚ IA and IB for blood type.
- Determine the genotypes of the parents⁚ For instance‚ a parent with blood type A could have the genotype IAIA or IAi‚ while a parent with blood type B could have the genotype IBIB or IBi.
- Fill in the Punnett square⁚ Place the alleles from each parent’s gametes in the corresponding boxes.
- Analyze the results⁚ The Punnett square will show the possible genotypes and phenotypes of the offspring. If the parents have different blood types (e.g.‚ one with type A and one with type B)‚ their offspring could have the blood types AB‚ A‚ or B‚ depending on the specific alleles inherited.
Codominance is an important concept in genetics as it demonstrates that multiple alleles can be expressed simultaneously‚ leading to diverse phenotypes. Recognizing codominance is crucial for understanding the inheritance of traits like blood type‚ flower color‚ and certain diseases.
Sex-Linked Traits
Sex-linked traits are characteristics determined by genes located on the sex chromosomes‚ specifically the X chromosome. These genes are not found on the Y chromosome‚ making males more susceptible to inheriting recessive sex-linked traits. This is because males only have one X chromosome‚ so if they inherit a recessive allele on that chromosome‚ they will express the trait. Females‚ with two X chromosomes‚ are less likely to express recessive sex-linked traits because they have a second X chromosome that could carry the dominant allele.
Examples of sex-linked traits include⁚
- Colorblindness⁚ This condition prevents individuals from distinguishing certain colors‚ usually red and green. The gene for normal color vision is dominant‚ while the gene for colorblindness is recessive.
- Hemophilia⁚ This disorder affects blood clotting‚ leading to excessive bleeding. The gene for normal blood clotting is dominant‚ while the gene for hemophilia is recessive.
- Duchenne muscular dystrophy⁚ This genetic disease causes progressive muscle weakness and degeneration. The gene for normal muscle function is dominant‚ while the gene for Duchenne muscular dystrophy is recessive.
To solve genetics problems involving sex-linked traits‚ it’s crucial to understand the difference in inheritance patterns between males and females. Punnett squares are still used‚ but the X and Y chromosomes must be included to represent the sex of the offspring. This allows you to predict the probability of offspring inheriting the trait‚ considering the genotypes of both parents and the sex of the offspring.
Pedigree Analysis
Pedigree analysis is a powerful tool used in genetics to trace the inheritance of traits through generations of a family. It involves constructing a diagram called a pedigree‚ which represents the family relationships and the presence or absence of a specific trait in each individual. Pedigrees are often used to study the inheritance of genetic disorders‚ but they can also be used to analyze the inheritance of any trait.
A pedigree typically uses standardized symbols to represent individuals and their relationships⁚ circles represent females‚ squares represent males‚ shaded symbols indicate individuals expressing the trait‚ and unshaded symbols indicate individuals without the trait. Lines connect individuals to show parent-offspring relationships and sibling relationships.
By analyzing the patterns of inheritance in a pedigree‚ geneticists can deduce the mode of inheritance for the trait in question. For example‚ if a trait appears in every generation and affects both males and females equally‚ it suggests an autosomal dominant inheritance pattern. Conversely‚ if a trait skips generations and affects males more frequently than females‚ it may indicate an X-linked recessive inheritance pattern.
Practice problems involving pedigrees often require students to determine the genotypes of individuals based on their phenotypes and family relationships. They may also ask students to predict the probability of offspring inheriting the trait based on the genotypes of their parents. Pedigree analysis is a valuable skill for understanding the transmission of genetic information across generations and for identifying the genetic basis of various traits and disorders.
Solving Genetics Problems
Solving genetics problems requires a systematic approach to unravel the inheritance patterns of traits. While the complexity of genetics problems can vary‚ a consistent set of steps can help you navigate through them effectively.
Firstly‚ carefully read and understand the problem statement. Identify the trait in question‚ the genotypes of the parents‚ and any other relevant information provided. Next‚ determine the possible gametes each parent can produce. This involves considering the alleles they carry for the trait and the possible combinations they can pass down to their offspring.
Then‚ set up a Punnett square‚ a diagram that helps visualize the possible genotypes of the offspring. The rows and columns of the Punnett square represent the gametes from each parent‚ and the squares within the grid show the resulting genotypes of their offspring.
Once you have filled out the Punnett square‚ determine the phenotypic ratios. This means calculating the percentage of offspring that will express each phenotype‚ based on the genotypes you have identified. Finally‚ answer the specific questions posed in the problem. This may involve calculating probabilities‚ predicting offspring phenotypes‚ or explaining the inheritance pattern of the trait.
Remember to practice consistently‚ and refer to resources like textbooks and online tutorials for additional support. With practice and a clear understanding of the principles of genetics‚ you will be able to confidently tackle even the most challenging genetics problems.
Comments (0)